Introduction
Many different species of plants produce triterpenoid saponins as part of their life cycle program during growth and development. Triterpenoid saponins are large groups of secondary metabolites that exhibit great structural diversity and a wide range of biological activities [1–3]. These compounds, such as ginsenosides, are commercially high-valued owing to their applications in medicine. The triterpene saponins in Panax ginseng Meyer, referred to as ginsenosides, have been especially noted as active compounds contributing to the efficacy of various pharmacological products [1]. Ginsenosides are synthesized via the isoprenoid pathway by the cyclization of 2,3-oxidosqualene to give primarily oleanane or dammarene triterpenoid skeletons. The basic backbone for the majority of triterpenoid saponins is derived from active isoprene units such as isopentenyl pyrophosphate (IPP) and its isomer, dimethylallyl pyrophosphate (DMAPP) before the cyclization step (Fig. 1). In higher plants, it was revealed that two pathways are involved in the biosynthesis of the IPP and DMAPP isoprene units. In the case of ginsenoside production, blocking the mevalonic acid (MVA) or 2-C-methyl-D-erythritol 4-phosphate (MEP) biosynthetic pathways by inhibiting the key enzymes will shed light on which pathway plays the most active role and how the two independent pathways are cross-regulated via pathway flux perturbation.
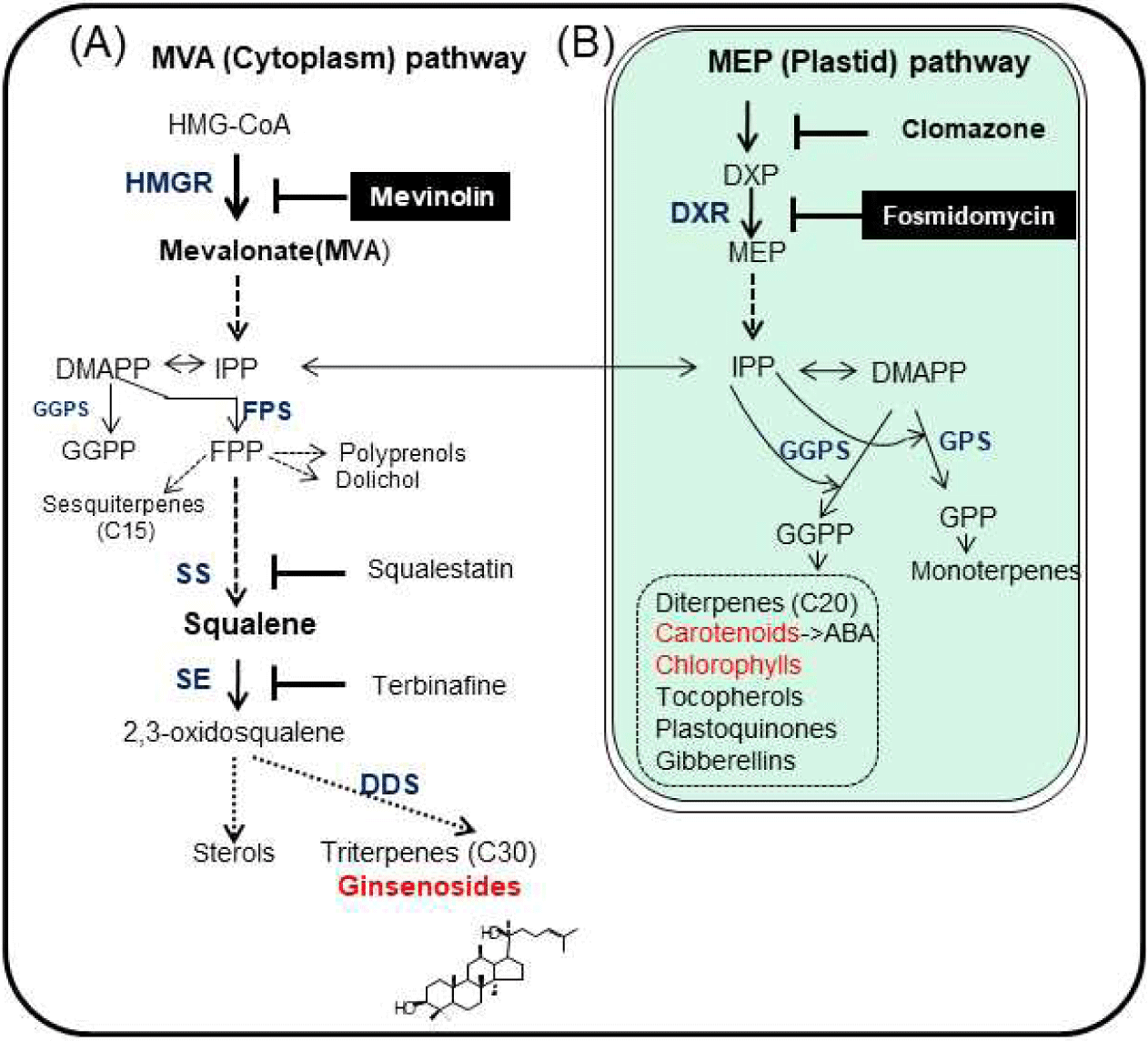
Mevinolin blocks the conversion of 3-hydroxy-3-methylglutaryl Co-A (HMG-CoA) into MVA by competitively inhibiting the binding of the substrate HMG-CoA to the active site of the enzyme HMG-CoA reductase (HMGR), and consequently blocks the synthesis of cytosolic IPP [4,5] and phytosterol biosynthesis [5,6] (Fig. 1). Mevinolin (10 μM) treatment for up to 7 days was previously reported to result in a reduction in ginsenoside production, and overexpression of the target gene of mevinolin, HMGR, resulting in increased biosynthesis of ginsenosides as well as phytosterols in transgenic ginseng adventitious roots by constitutive overexpression of ginseng HMGR [7]. Fosmidomycin inhibits the production of MEP from 1-deoxy-d-xylulose 5-phosphate (DXP) [8,9] by competitively inhibiting 1-deoxy-D-xylulose-5-phosphate reductoisomerase (DXR). Different concentrations of inhibitors and the duration of incubation can affect the inhibition rate and scale. However, treatment with less than 50 μM of mevinolin did not alter the growth rate of ginseng hairy roots and reduced the amount of three ginsenosides, Rb1, Re, and Rg1 [10].
In this study, inhibition isoprenoid pathways were analyzed for the production of seven ginsenosides, Rg1, Re, Rg2, Rb1, Rc, Rb2, and Rd using adventitious roots generated from P. ginseng Meyer to elucidate the major roles of the MVA and MEP pathways. In addition, the inhibitory effect of the MVA pathway on isoprenoid-derived products biosynthesized via the MEP pathway was analyzed.
Materials and Methods
Ginseng adventitious roots generated from P. ginseng Meyer were obtained from Magoplants (Korea) and continuously sub-cultured on liquid B5 media (Duchefa Biocheme, The Netherlands) with 3% sucrose and 2 mg/L indole-3-butyric acid, which was replaced every 5 weeks. The Columbia ecotype (CS 60000) of Arabidopsis thaliana was used in this study. Seeds were surface sterilized and sown on 1/2 MS (Duchefa Biocheme) media containing 1% sucrose, 0.5 g/L MES (2-[N-morpholino]ethanesulphonic acid) and 0.8% phytoagar at pH 5.7 with KOH. Three-day cold-treated seeds were germinated under long-day conditions of 16 h light/8 h dark at 23℃.
Mevinolin (Mev) was purchased from Sigma-Aldrich (USA) and fosmidomycin from Molecular Probes (USA). Stock solutions of Mev (10 mM) in ethanol and fosmidomycin (100 mM) in distilled water were stored at –20℃. For inhibitor treatment of ginseng adventitious roots, after 4 weeks of pre-cultivation, 10 µM Mev or 100 μM fosmidomycin was added to an Erlenmeyer flask on a shaking chamber at 23℃. After 3 days of treatment, harvested adventitious roots were frozen for ginsenoside analysis.
For the analysis of ginsenosides from adventitious roots, milled powder (0.2 g) was soaked in 80% MeOH at 70℃. After evaporation, the residue was dissolved in distilled water (H2O), followed by extraction with H2O-saturated n-butanol. The butanol layer was evaporated to produce the saponin fraction. Each sample was dissolved in MeOH (1 g/5 mL), filtered through a filter (0.45 µm), and used for HPLC analysis. The HPLC separation was carried out on an Agilent 1260 series HPLC system (USA). The sample was detected at a wavelength of 203 nm by UV-spectrometry. Quantitative analysis was performed with a one-point curve method using external standards of authentic ginsenosides.
Chlorophyll and carotenoids were extracted from 50 mg of frozen tissue by homogenization in cold 80% acetone (10 mg/1 mL). The concentration of total chlorophylls and carotenoids were determined by spectral measurements as described previously [11] by using a microtiter plate reader (BioTek Instruments, ELx800 Absorbance Microplate Reader) at an absorbance of 480, 645, and 663 nm.
Results and Discussion
In order to understand the major pathways involved in ginsenosides biosynthesis, inhibitors were tested using adventitious roots generated from P. ginseng Meyer. Triterpenoid ginsenosides are normally biosynthesized from two isoprenoid pathways, the cytoplasmic MVA and plastidial MEP pathways (Fig. 1). The activity of the enzymes that catalyze the first committed steps in the MVA and MEP pathways has been shown to be blocked by the specific inhibitors mevinolin and fosmidomycin. To avoid non-specific ginsenosides and exclude already biosynthesized ginsenosides, newly emerged root tips were subcultured with inhibitors. Depending on different sets of adventitious roots, small fluctuations of individual ginsenoside content could not be excluded. Mevinolin treatment resulted in reduced total ginsenosides and the content of its seven related major ginsenosides (Rg1, Re, Rg2, Rb1, Rc, Rb2, and Rd) (Fig. 2). It reduced ginsenoside content by up to 36% in total (Fig. 2A) and the greatest reduction was in Rb1, which was reduced by 55% (Fig. 2B). Total ginsenoside reduction was similar to previously observed results using hairy roots [10]; however, it is interesting to note that the Re reduced the highest in hairy roots. The starting materials, that is, the parts or organs, as well as the ginseng cultivars used for the generation of hairy or adventitious roots, could be factors contributing to the modulation of individual ginsenoside levels.
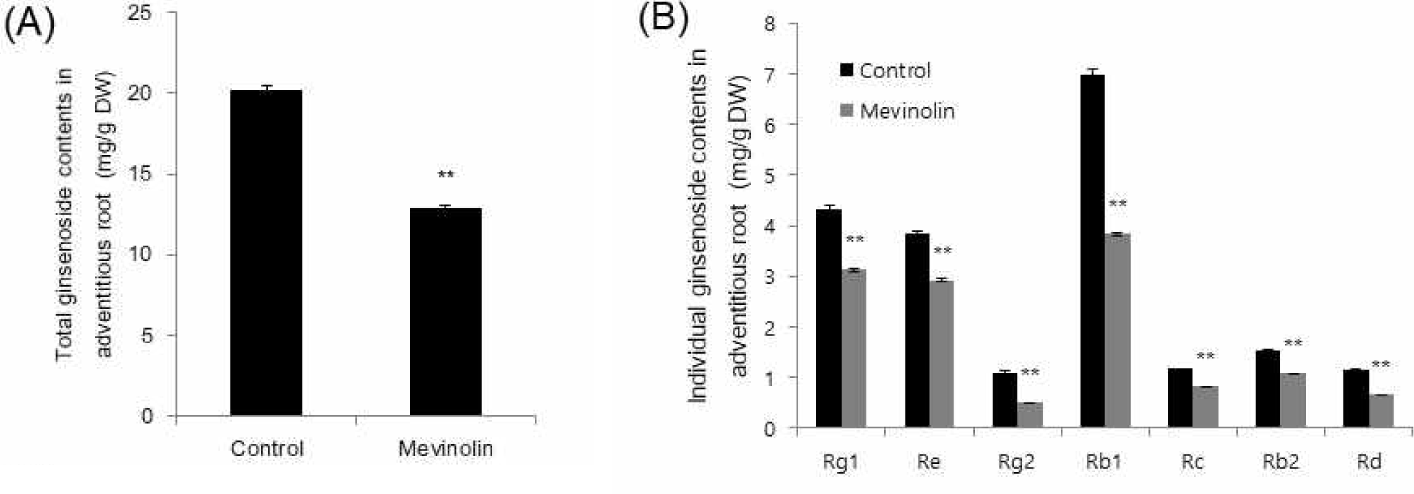
Fosmidomycin treatment also resulted in reduced total ginsenoside content in two different biological repeats (Fig. 3A and 3C). The corresponding individual ginsenosides showed few differences. When Rb1 is reduced, Rd is significantly increased and vice versa (Fig. 3B and 3D). Depending on the specific regions of roots or incubation times of inhibition, individual ginsenoside content can be affected. However, it is clear that the total ginsenoside is reduced by up to 13.2% and 6.05% (Fig. 3A and 3C respectively). Similar reduction was also observed in ginseng hairy roots focused on only three ginsenosides, Rb1, Re, and Rg1 [10]. Notably, the reduction rate was also higher as a result of mevinolin treatment than as a result of fosmidomycin treatment [10]. These results suggest that the MVA pathway in root tissues, might play a more important role in triterpene ginsenoside production than the MEP pathway. It is also considerable that the other studies have reported where the MEP pathway to be more dominant in the ginsenoside pathway within P. ginseng leaves [12].
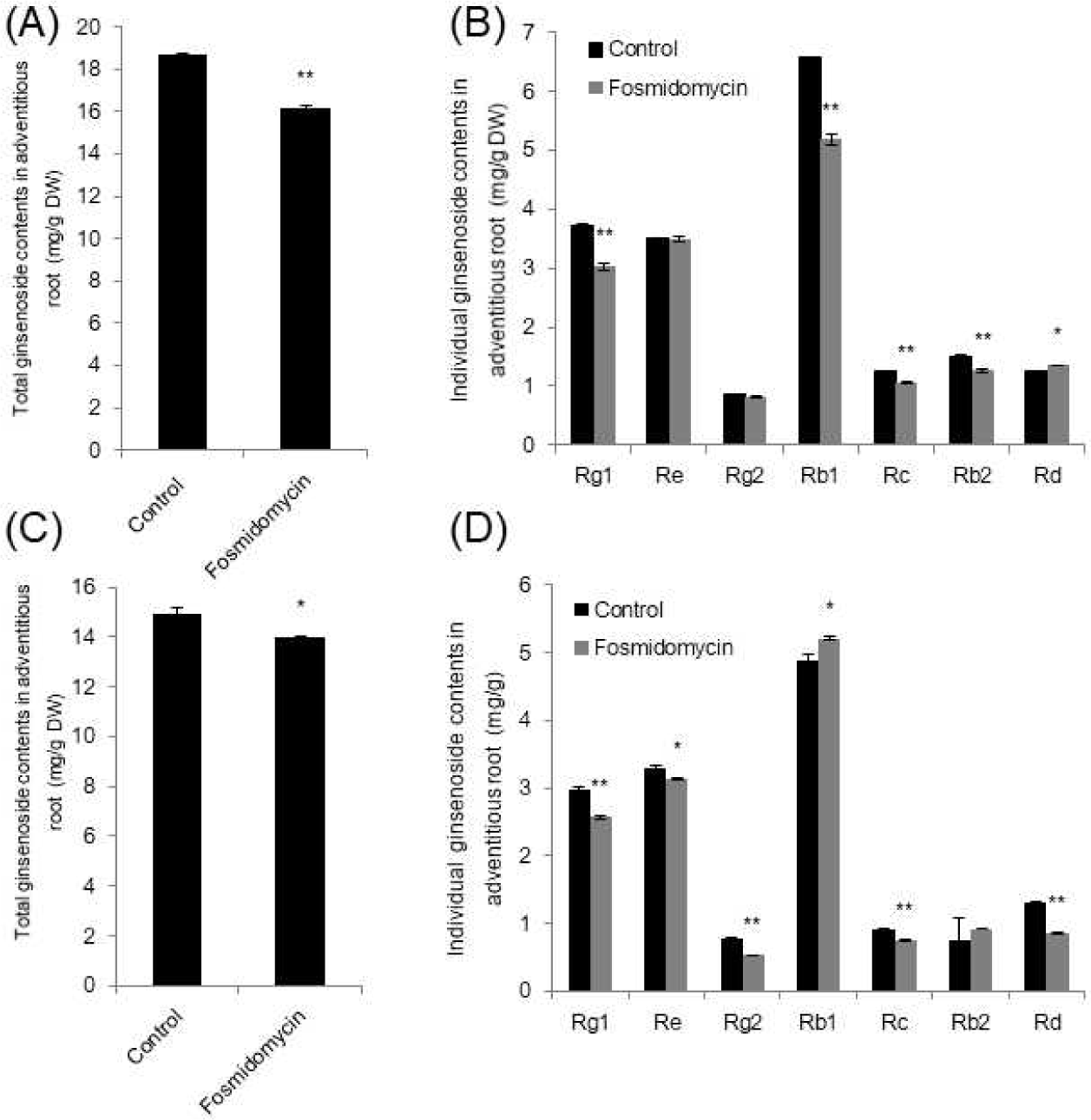
Total plant sterols such as campesterol, sigmasterol and sitosterol are increased up to 2.2-fold via enhancement of the MVA pathway [13]. Enhancement of the MVA pathway was observed by mevalonate (MVA) treatment on growth media. In order to understand whether the triterpene ginsenosides are also increased by MVA application, 5 mM of MVA was added in 1/2 MS liquid adventitious root culture media and grown for an additional three days. Locations of the changes in ginsenoside content over the 3 days were analyzed, and the total amount of major ginsenosides did not differ from that of the control (Fig. 4A). However, individual ginsenosides fluctuated. Rg1, Rc, Rb2, and Rd significantly increased, whereas Re and Rg2 decreased (Fig. 4B). The content of the most abundant ginsenoside in adventitious roots, Rb1, was not altered (Fig. 4B). The results suggest that the additional supply of MVA does not change total ginsenoside content, which indicates that the individual ginsenosides keep certain amounts of cytotoxic metabolites via homeostasis mechanisms. This strongly suggests that the post-translational regulation of rate-limiting HMGR genes is involved [7].
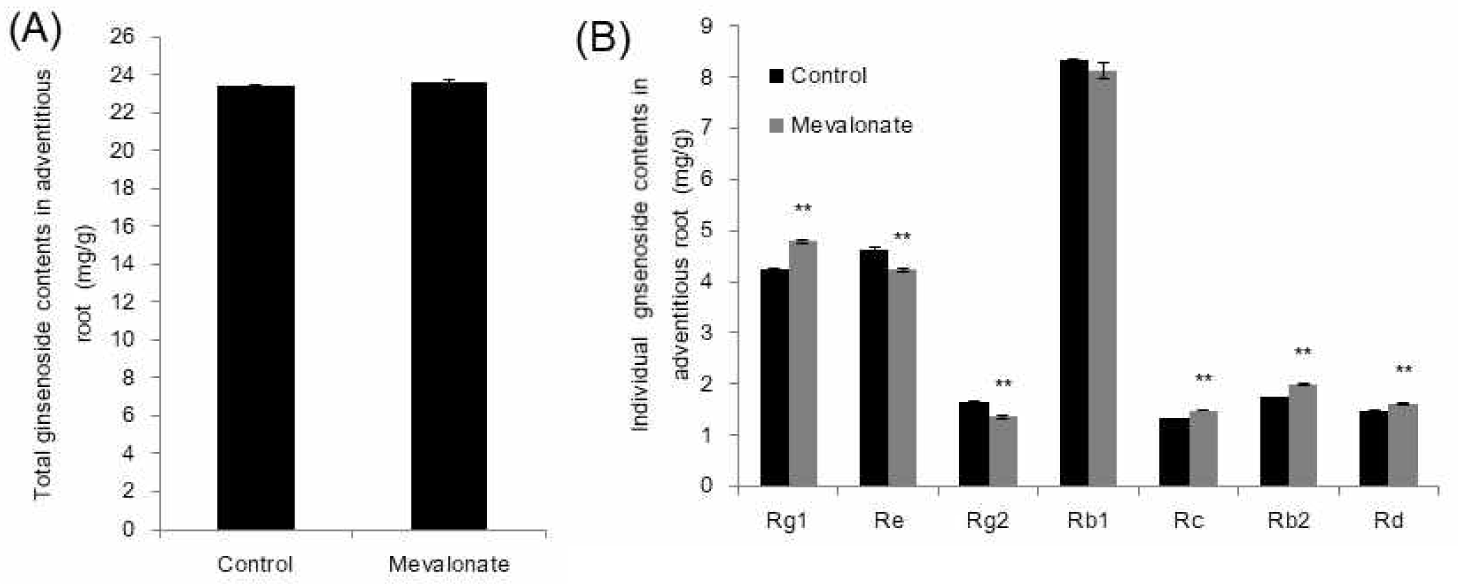
Cross-talk between the cytosolic mevalonate and the plastidial MEP pathways has been reported [14,15]. To observe the flux influence of MVA pathway perturbation on the MEP pathway, Arabidopsis seedlings were treated for 4 days with mevinolin, because this is also applicable to ginseng [7]. Chlorophylls and carotenoids are biosynthesized via the MEP pathway (Fig. 1) as well as other secondary metabolites. Mevinolin treatment caused a 10% increase in chlorophyll a and resulted in total chlorophyll increase (Fig. 5A). MVA inhibition by mevinolin also increased carotenoid content (Fig. 5B). As expected, fosmidomycin, which inhibits the plastidial MEP pathway, caused 47% and 43% reductions in chlorophyll a and carotenoid content, respectively (Fig. 5). This result supports the hypothesis that a possible transport mechanism of IPP between the two pathways exists. In accordance with these results, an isoprene transport assay of ginsenoside biosynthesis is needed for future evaluation.
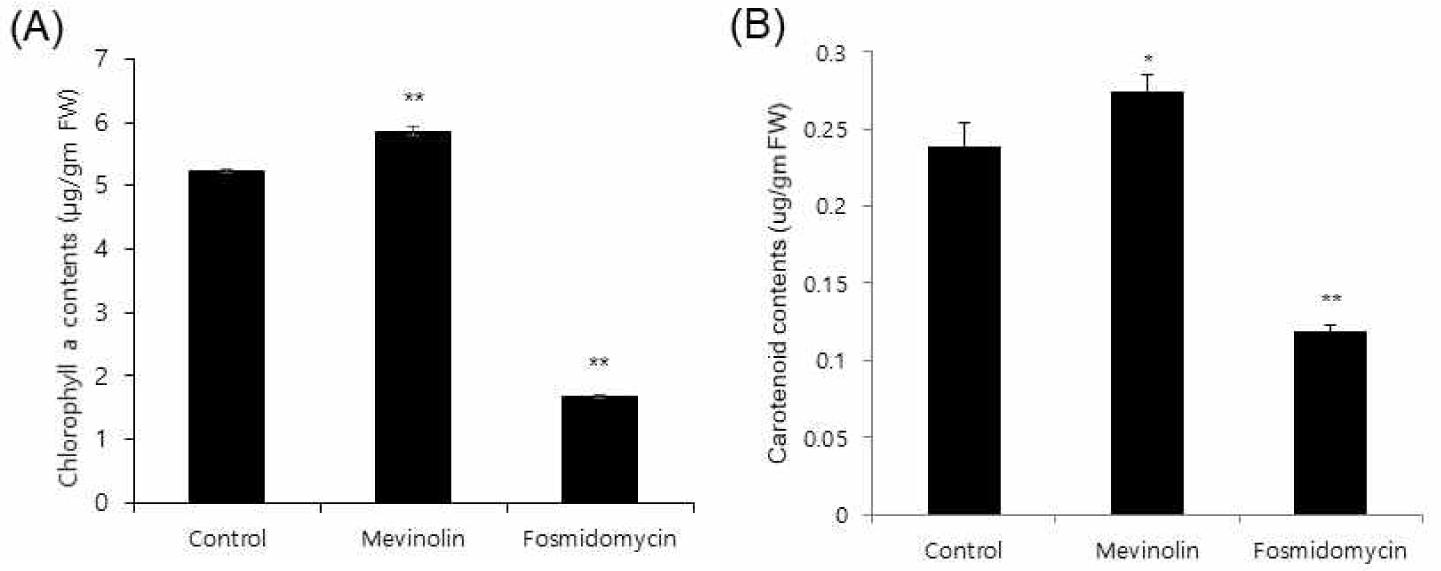
Conclusion
The universal precursor of ginsenoside biosynthesis, isopentenyl diphosphate (IPP), is biosynthesized by the utilization of two pathways. IPP and its isomer dimethylallyl diphosphate (DMAPP) are so-called the five-carbon isoprene basic units. In higher plants, it has been demonstrated that the cytoplasmic MVA pathway and the later discovered MEP pathway are involved in the biosynthesis of the active isoprene unit [14]. The existence of natural inhibitors in both pathways provides a valuable tool for the study of ginsenoside biosynthesis. Mevinolin, also known as a lovastatin, is a competitive inhibitor of the key enzyme 3-hydroxy-3-methylglutaryl-coenzyme a reductase (HMGR) in the MVA pathway. Fosmidomycin is a later identified inhibitor of the first committed enzyme, 1-deoxy-D-xylulose-5-phosphate reductoisomerase, of the plastidial MEP pathway. With such inhibitors at hand, it is possible to deregulate the biosynthesis pathway and ultimately deplete metabolite production from treated cells. Our study was therefore aimed at elucidating the main pathway of ginsenosides biosynthesis in P. ginseng by inhibition of the MVA, and MEP pathways. Inhibition of the MVA pathway by mevinolin for 3 days decreased total ginsenoside content by up to 36%, affecting all 7 major ginsenoside monomers tested (Fig. 2). Modulated ginsenoside content is observed from the 1st day of treatment, which is greater than 3 days of treatment, and start recovering within 7 days [7]. Depending on the inhibitor concentrations and incubation times, the rate of decrease can be even greater. Fosmidomycin also inhibited total ginsenoside production at a lesser strength when tested at a concentration of 100 μM (Fig. 3). Depending on the adventitious root age and conditions, the decrease rate is different. However, total ginsenosides reduction by fosmidomycin inhibition was not more than 15% (Fig. 3). This indicates that the MVA pathway may play a more important role than the MEP pathway in the production of ginsenosides. Interestingly, exogenous MVA supply did not alter final total ginsenosides although individual ginsenoside monomers fluctuated (Fig. 4). This can be explained by the posttranslational modification of rate-limiting enzymes such as HMGR [7]. Endogenous MVA production is tightly regulated at many levels. Thus, supply of MVA seems to activate a feedback regulatory mechanism of MVA pathway genes. Continuous inhibition of the MVA pathway stimulated the MEP pathway and resulted in an increase in metabolites such as chlorophyll and carotenoids (Fig. 5). This indicates that the cross interaction between the MVA and MEP pathways in plants are tightly regulated. Based on these results, genetic engineering in ginsenoside production using commercially available ginseng adventitious roots should consider both the MVA and MEP pathways.