Introduction
Fluorescent pseudomonads in the rhizosphere are noted for their plant-benefiting traits, including inhibition of root-pathogens, induction of systemic resistance in plants against biotic and abiotic stress, and solubilization of mineral nutrients [1–4]. As they control plant diseases, several plant growth-promoting pseudomonads are formulated as bioinoculants [5–9]. The mechanisms involved in promoting plant growth upon root colonization by pseudomonads include the production of plant hormones, modulation of ethylene by ACC deaminase, and solubilization of soil minerals, such as phosphate and iron [2,10]. Despite improving plant health, the metabolites and the mechanisms involved in pseudomonad-mediated plant growth and morphology are obscure.
Some microbial metabolites such as hydrogen cyanide (HCN) and phenazines exert negative effects on plant growth [11,12], whereas other volatiles promote plant health. Many plant growth-promoting Bacillus strains produce organic volatiles, such as 2R, 3R-butanediol, which enhance plant growth and induce disease resistance [13–15]. In butanediol-mediated plant growth, signaling occurs through cytokines [14]. Conversely, the ethylene signaling pathway operates via the butanediol-mediated induced systemic resistance [13]. A recent study indicated that 2R, 3R-butanediol produced by beneficial bacteria triggers the secretion of root exudates that attract rhizosphere bacteria and fungi, thereby promoting root colonization [16].
The production of 2R, 3R-butanediol by P. chlororaphis O6 is controlled by the GacS/GacA global regulatory system [17–19]. This compound confers protection against drought in plants, and this protective effect correlates with abscisic acid-independent stomatal closure and nitric oxide- and hydrogen peroxide-mediated gene expression changes [18,20–22]. Increased levels of the osmolyte galactinol occurs with colonization of A. thaliana roots by P. chlororaphis O6 [23,24].
HCN is another volatile produced by P. chlororaphis O6 and other pseudomonads; it is involved in the control of insects and nematodes [25,26]. The inhibition of metal-containing enzymes in the respiratory electron transport chain of these organisms by HCN may be key in its activity [27]. HCN also affects plant growth [11,28].
This paper addresses how volatiles, including HCN, produced by P. chlororaphis O6 alter the growth of the model plant A. thaliana and how bacterial growth media affect volatile composition. The work focused on changes in root morphology as well as examination of leaf mass production, which have previously been used as a measure of plant growth promotion [13–15]. We anticipated that the absence of the volatile, butanediol, in the gacS mutant would eliminate plant growth promotion. However, the role of HCN, which is also produced under GacS control, as another active volatile from P. chlororaphis O6 was explored by comparing plant growth effects with a gacS mutant defective in HCN. This was done through an insertion in the hcnA gene, which is required for HCN production [26]. The pathways in the plants responsive to P. chlororaphis O6 volatiles were studied using three A. thaliana mutants—one was defective in reception of ethylene (etr1), another lacked sensitivity to jasmonic acid (coi1-1) and the third (NahG) could not accumulate salicylic acid (SA). Our findings suggest that more than one volatile is important in modifying plant growth and that variability in the responses could be because of the effects of the bacterial nutrient medium on the production of volatiles.
Materials and Methods
Wild-type P. chlororaphis O6 [29], a previously constructed gacS mutant, the complemented gacS mutant [30], and an hcnA mutant [26] were used in this study. For each experiment, 15% glycerol stocks (stored at −80°C) of P. chlororaphis O6 strains were streaked on nutrient agar (NA; Difco, Detroit, MI, USA) plates supplemented with streptomycin (50 μg/mL) and ampicillin (50 μg/mL), kanamycin (50 μg/mL), or tetracycline (25 μg/mL) depending on the strain and incubated at 25°C for 2 d. These cultures were used to inoculate 10 mL of nutrient broth or Methyl Red Vogues Proskauer (MR-VP; Sigma-Aldrich, St. Louis, MO, USA). The MR-VP medium was used, because it promotes butanediol production and no phytotoxic effects of volatiles were reported when other bacterial cells were grown in this medium [31]. For liquid culture, the bacterial cultures were incubated overnight in a rotary shaker (180 rpm) at 25°C. Undiluted bacterial cultures were used in the direct application experiment.
Wild-type A. thaliana ecotype Columbia (Col-0) was obtained from the Ohio State University Stock Center (Ohio State University, Columbus, OH, USA). The mutant lines used were etr1 (insensitive to ethylene) [32], coi1-1 (insensitive to methyl jasmonate) [33], and NahG (transgenic line that degrades SA) [34].
A. thaliana seeds were sterilized using 70% ethanol and 2% sodium hypochlorite solution for 10 min, followed by washing five times with sterile water as described previously [35]. The sterilized seeds were placed on Murashige and Skoog (MS, pH 5.7; Sigma-Aldrich) medium supplemented with 0.25% (w/v) Phytagel and 2% (w/v) sucrose [36] in Petri dishes (SPL Life Science, Pocheon, Korea). The plants were grown in a growth room for 2 weeks with 8 h darkness and 16 h light cycle (200 μmol photons m–2 s–1). The temperature was maintained at 22±2°C with a relative humidity of 50%–60%.
Two weeks after germination and growth on MS medium, three A. thaliana seedlings of similar size and growth stage were transplanted to one side of an I-plate (87.5×15 mm2, SPL Life Science) containing MS solid medium (10 mL). The other side of the I-plate contained NA or MR-VP agar (10 mL) to grow P. chlororaphis O6 strains. An inoculum made of a 20 μL suspension (OD600=0.1, approximately 1×108 CFU/mL) of P. chlororaphis O6 cells was grown on NA plates, washed and suspended in sterile water, and was then applied to the NA or MR-VP agar. Aliquots (20 μL) of sterile bacterial culture medium were used as the control treatment. The inoculated I-plates were sealed with parafilm, and the plants were allowed to grow for 2 weeks. After incubation, the growth parameters of A. thaliana were measured; root development was observed using a M165 FC microscope (Leica Microsystems, Tokyo, Japan).
In a second experimental setup, MR-VP or NA agar was poured into one well of a 12-well cell culture plate (well dimension 21.9 mm×17.5 mm, SPL), and the remaining wells were filled with MS medium. Wild-type P. chlororaphis O6 suspension (10 μL) was inoculated into the well containing the bacterial growth media, and A. thaliana Col-0 seedlings were transplanted to rest of the wells containing the MS medium. Consequently, all plants in one plate were exposed to the volatiles from P. chlororaphis O6. The plates were sealed firmly with parafilm and placed in the plant growth room. The experiment was repeated twice each with three replicates. The images of the plants were captured 2 weeks after incubation.
To determine the effect of the treatments on A. thaliana, each plant was removed from the I-plate 2 weeks after incubation and the roots were excised from the shoot. Excess moisture was removed from the leaves using paper tissues, and the shoots were immediately weighed using an analytical balance (A&D Korea, Seoul, Korea). Root lengths were measured using a digital ruler (Mitutoyo, Kanagawa, Japan). The experiment was repeated twice with three plants.
Results
In the I-plate and 12-well agar plate bioassays, the growth effects of the volatiles produced by wild-type P. chlororaphis O6 on A. thaliana Col-0 differed with varying growth media (Fig. 1 for the I-plate assays and Fig. 2 for the well plate assays). In the I-plate with NA as the bacterial growth medium, volatiles from wild-type P. chlororaphis O6 significantly inhibited root development, as measured by root branching and elongation, and decreased shoot growth (p<0.0001; Fig. 3). Similar changes to root growth were seen with two other rich growth media, namely Kings medium B and Lysogeny Broth medium (data not shown). However, volatiles produced from the wild type on the MR-VP medium caused shortening of the roots, while promoting extensive lateral branching (Fig. 1). There was little visual difference in the growth of plants exposed to volatiles from the gacS mutant from that of the controls on either bacterial growth medium (Fig. 1). The resulting measurements for root length and branch numbers, influenced by the volatiles produced during NA growth, are shown in Fig. 3. The data confirmed the visual findings of inhibited root elongation by the volatiles from the wild-type strain but not with those from the gacS mutant. Strong inhibition of root elongation by volatiles was restored when the gacS mutant was complemented. The number of lateral branches for plants exposed to the volatiles from the gacS mutant was significantly lower than that for the controls; however, it was higher than that for plants exposed to volatiles from the wild type or complemented gacS mutant (Fig. 3). These findings suggest that the inhibitory microbial volatiles are under GacS control in P. chlororaphis O6.
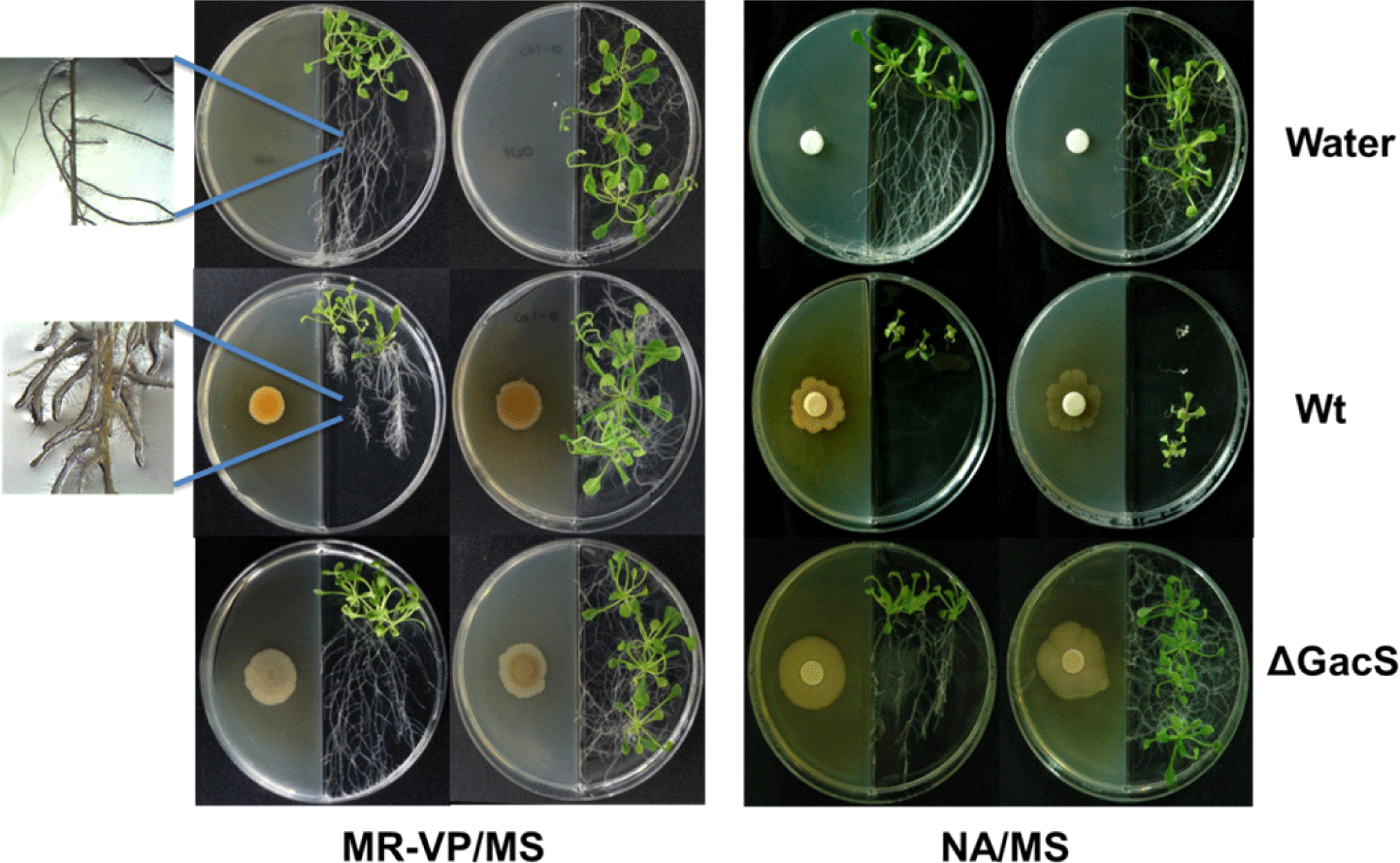
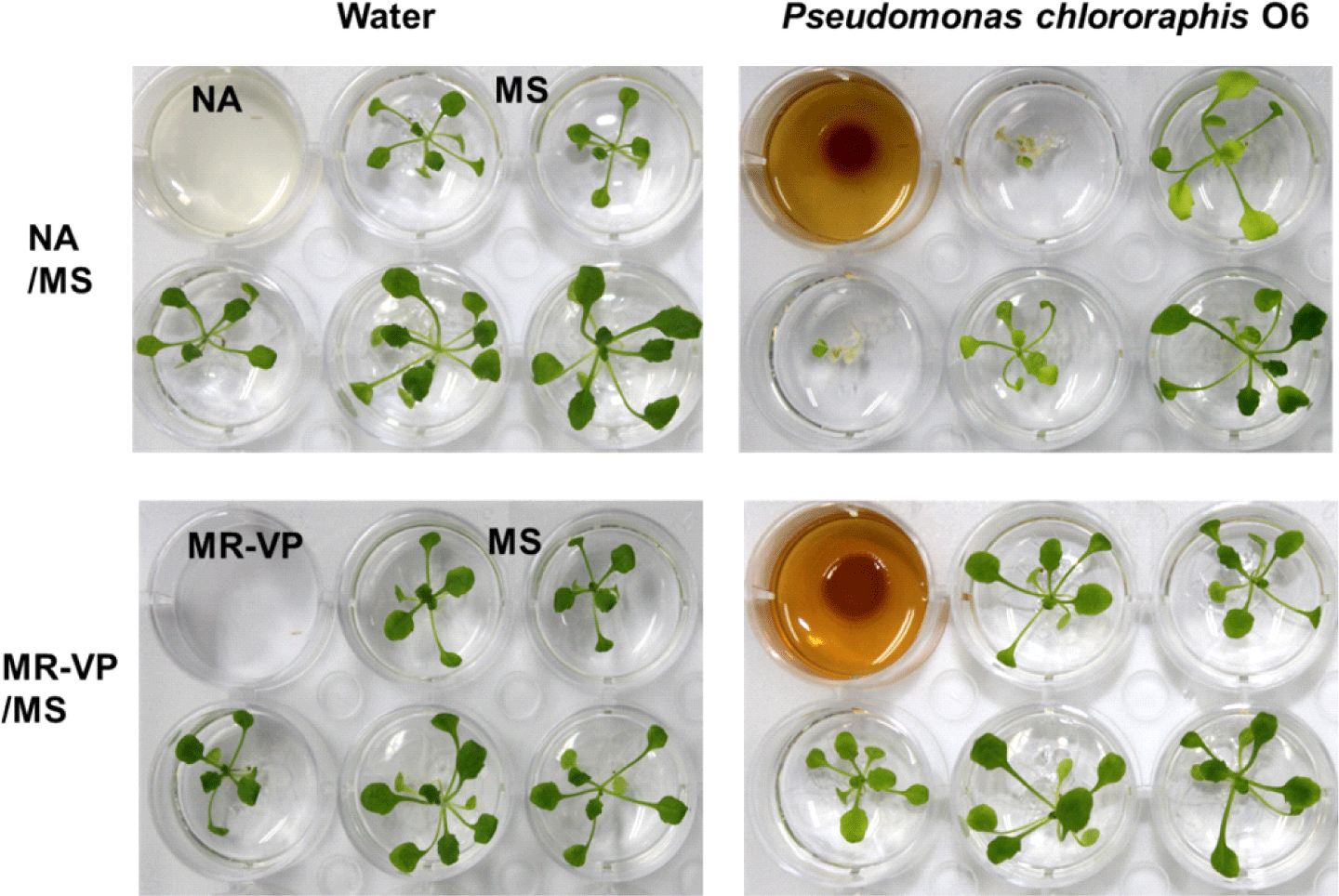
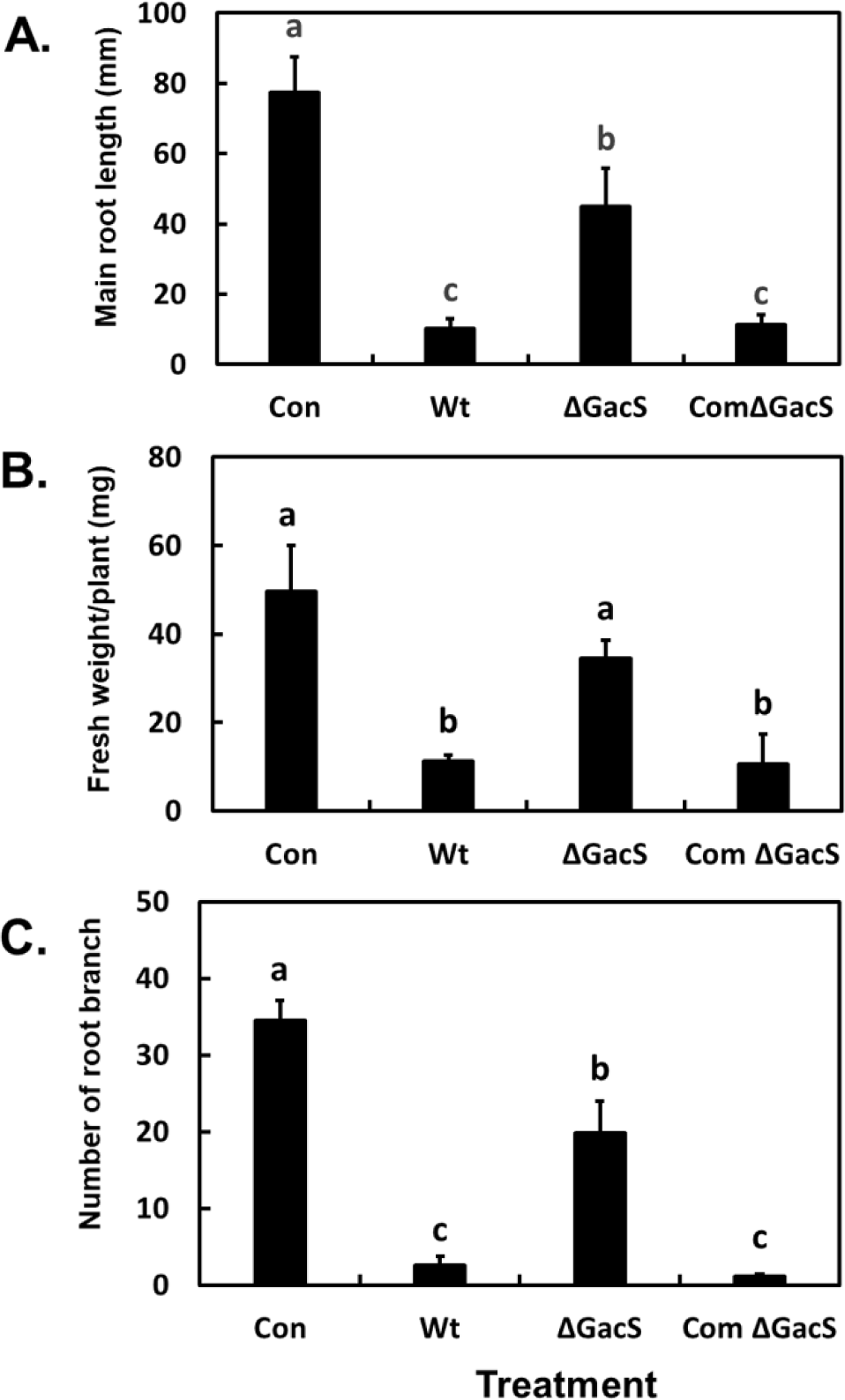
Data for the effect of the volatiles on root morphology when the bacteria were grown on MR-VP agar are shown in Fig. 4. The lateral and main root lengths of A. thaliana Col-0 exposed to volatiles from the wild-type P. chlororaphis O6 strain were significantly lower than those of control plants (p<0.0001); however, the level of reduction was less than that with volatiles from bacterial growth on the NA agar (Fig. 3). The volatiles produced from wild-type bacterial growth on MR-VP agar greatly increased root branching (Fig. 1 and Fig. 4). These effects of the volatiles on root morphology were dependent on a functional GacS system. Root morphology and development of A. thaliana were similar to those of control plants between plants exposure to the volatiles from the gacS mutant grown on the MR-VP medium (Fig. 4).
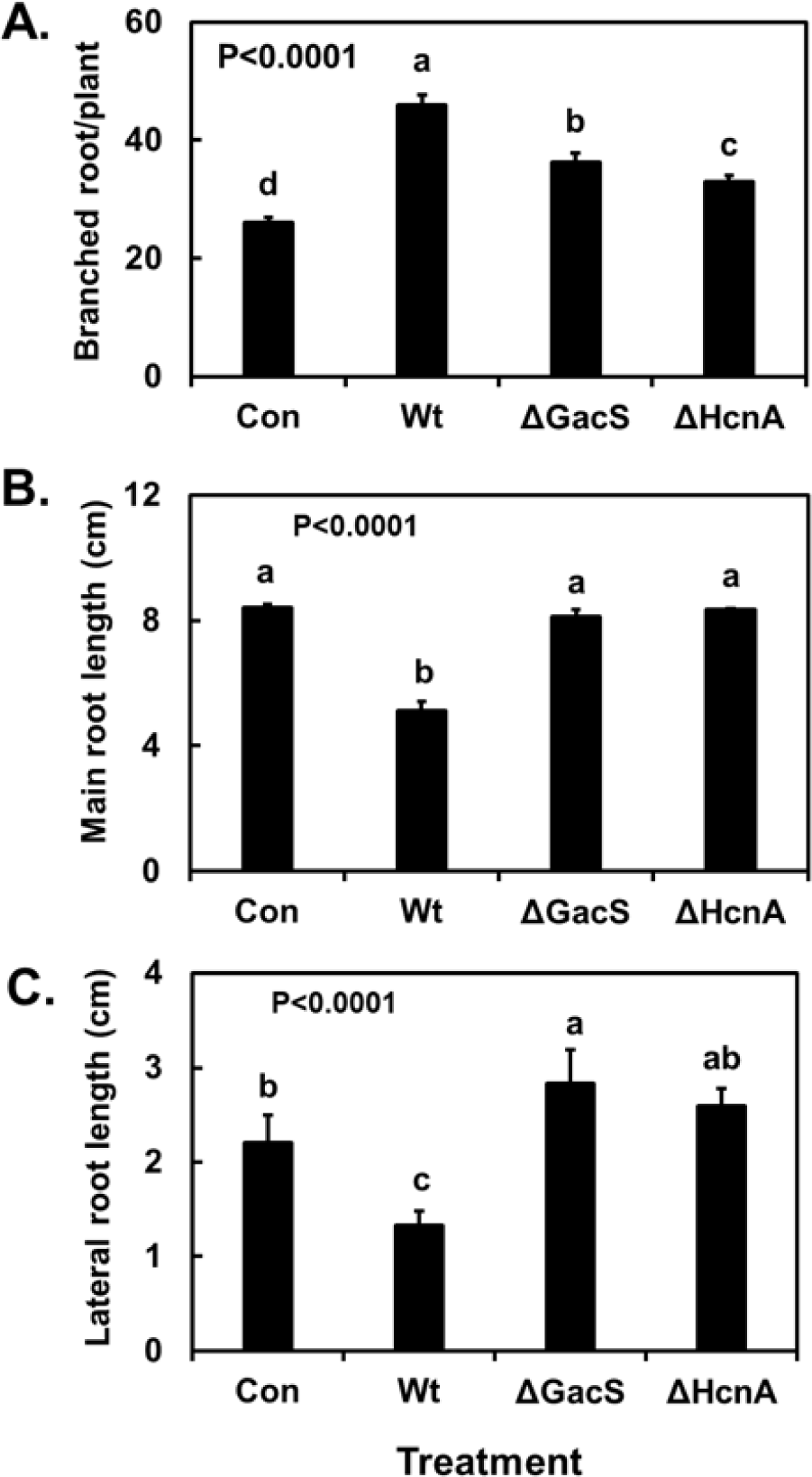
Many metabolites from P. chlororaphis O6 are affected in a gacS mutant. Two known volatiles under GacS control are butanediol and HCN. Growth on MR-VP medium with volatiles from the hcnA mutant, which was unable to produce HCN, showed root growth similar to that of control plants not exposed to microbial volatiles, but there is an exception for root branching (Fig. 4). Root branching was decreased for plants exposed to the volatiles lacking HCN but was still higher than that of control plants (Fig. 4). However, elongation of the main root and laterals was significantly greater with the volatiles lacking HCN than with volatiles from the wild-type strain (Fig. 4). The results obtained from volatiles of the hcnA mutant indicate that HCN was among the active volatiles affecting A. thaliana growth.
Results of changes in leaf development because of exposure to microbial volatiles when grown on MR-VP medium are shown in Fig. 5. With the wild-type plant (Col-0), the microbial volatiles from the wild-type strain stimulated leaf mass to a greater extent than those from the gacS mutant. Leaf masses of the coi1-1 and nahG mutants were similar to those of the Col-0 plants, suggesting that the jasmonic acid and SA pathways were not significant (Fig. 5). With regard to the microbial volatiles, leaf mass of the coi1-1 and nahG plant mutant lines was reduced with the volatiles from the wild-type strain but was stimulated by those from the gacS mutant. Thus, the jasmonic acid and SA pathways were required for response to the volatiles from the wild-type P. chlororaphis O6 that reduced leaf mass.
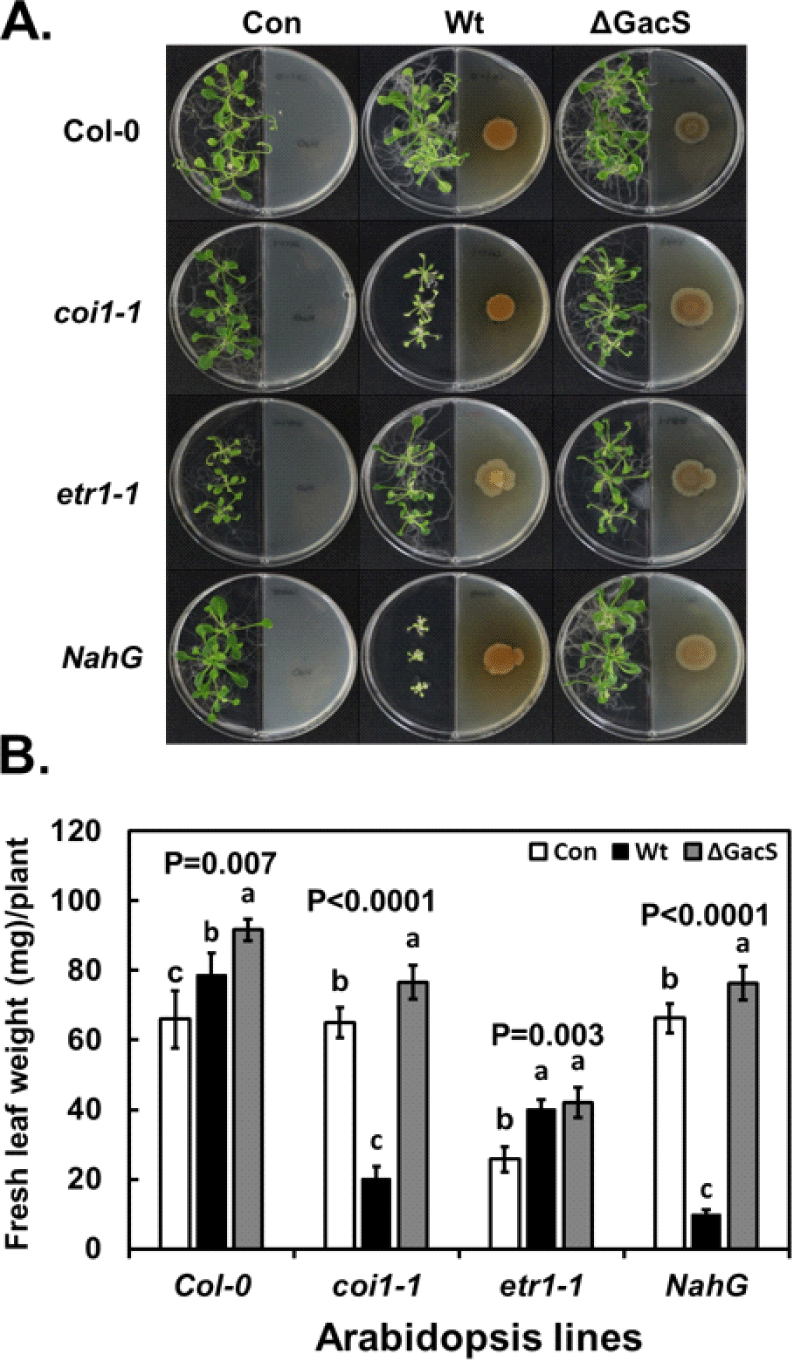
The response of the two mutant plant lines was similar to that observed in the wild-type plant when exposed to microbial volatiles produced on NA medium. The products from the wild type and complemented-gacS mutant inhibited leaf mass, but the volatiles from the gacS mutant increased leaf growth (Fig. 5). Reduced leaf mass was visibly observed in wild-type plants grown on NA medium with a marked gradient effect, which was dependent on the distance from the well exhibiting bacterial growth (Fig. 2). Leaf growth effects were not observed when MR-VP medium was used (Fig. 2).
The growth of etr1-1 mutant plants showed reduced leaf mass when grown in I-plates without microbial volatiles produced on MR-VP medium in comparison with that of the wild-type Col-0 strain (Fig. 5). However, leaf growth was improved with microbial volatiles from both the wild-type and gacS bacterial strains (Fig. 5). Thus, the pattern of changes in leaf mass in response to the microbial volatiles was similar to that of the wild-type Col-0 plant line, although overall growth was reduced in the plants lacking an intact ethylene response pathway. These findings show that irrespective of an ethylene-response pathway in the plant, the volatiles from P. chlororaphis O6 will improve leaf mass. These volatiles were not dependent on GacS regulation.
Discussion
This study illustrated that nutrition of a root-colonizing pseudomonad regulates the production of volatiles that alter the growth potential of A. thaliana. Both root morphology and leaf mass were sensitive to the microbial volatiles. Previous studies with fluorescent pseudomonads showed that HCN production was limited in MR-VP medium when compared with that in NA medium, whereas production of 2R, 3R-butanediol was enhanced in MR-VP medium [11,37]. Thus, NA growth of wild-type P. chlororaphis O6, with conditions that favored HCN production, generated volatiles that significantly inhibited leaf and root growth. Normal leaf and root growth upon exposure to volatiles that lack HCN from the gacS or hcnA mutants growing on NA was consistent with HCN playing an important role in the inducing inhibition. A plant growth study utilizing MS agar amended with KCN at 1,000 μg/mL—but not at 100 μg/ mL—on one side of I-plates revealed a volatile phytotoxic effect on A. thaliana that was growing on nonamended MS agar on the other side of the I-plate (data not shown). These findings confirmed the phytotoxicity of volatile HCN. Thus, this effect of HCN in inhibiting plant growth adds to its roles as a key nematicidal and aphicidal volatile from P. chlororaphis O6 [26,37]. The production of reactive oxygen species through alternative oxygenase 1 is proposed be a major mechanism for HCN toxicity [11].
The observed enhanced leaf growth in wild-type A. thaliana by volatiles produced by P. chlororaphis O6 growing on MR-VP medium was consistent with 2R, 3R-butanediol production by the wild-type strain. This bacterial volatile is documented to promote plant growth [14]. However, the increase in leaf mass with volatiles from the gacS strain, which would not produce 2R, 3R-butanediol, suggested that unidentified volatile microbial metabolites were involved.
The results of the growth assay with A. thaliana lines defective in the defense-signaling pathways differed upon exposure to P. chlororaphis O6 volatiles. Both functional jasmonic acid sensitivity and SA accumulation were required to eliminate reduced leaf mass caused by volatiles from wild-type P. chlororaphis O6 produced on MR-VP medium; however, ethylene sensitivity was not an influential factor. In wild-type A. thaliana Col-0, volatiles produced by both the wild type and gacS mutant of P. chlororaphis O6 promoted leaf mass. Although increased leaf rosette biomass and seed yield in SA-deficient nahG transgenic lines has been reported [38], we did not observe improved leaf mass under our growth conditions for the nahG line when compared with that for Col-0. Seed germination, photosynthesis, growth, respiration, and plant defense have been linked to SA production in other studies [39]. Jasmonic acid also functions in plant development and interacts with the cytokine signaling pathway [40,41]. Using A. thaliana lines defective in signal pathways, a role for cytokines in 2R, 3R-butanediol mediated-plant growth promotion is proposed [14].
In summary, our results indicate that volatiles produced by P. chlororaphis O6 has different effects on shoot and root plant growth depending on the bacterial nutrient conditions. The GacS/GacA regulatory system was important for the synthesis of the plant-active volatiles from P. chlororaphis O6. Currently, different roles for two microbial volatiles—HCN as an inhibitor and butanediol as a plant growth activator—have been identified; however, the study indicated the involvement of additional volatile metabolites. Potential benefits of volatiles affecting lateral root development include enhanced survival to environmental stress. The volatiles from P. chlororaphis O6 affected plant growth and development through the involvement of both the jasmonate and SA defense signaling pathways.