서 론
식물 엽록체는 cyanobacteria가 내부공생과정을 통하여 진핵세포로 도입되어서 확립된 세포소기관으로 알려져 있다(Zimorski et al., 2014). 하지만 cyanobacteria가 기존에 가지고 있던 대부분의 유전자들은 진핵세포의 핵으로 이동하였다. 그리고 90%가 넘는 대부분의 엽록체 단백질들은 핵에서 발현되고, 세포질에서 합성되어 엽록체로 이동하게 된다. 엽록체는 외막, 내막, 외막과 내막 사이의 intermembrane space, 내막 내부의 stroma, 그리고 stroma 내에 막으로 구성되어 있는 thylakoid라는 구조로 이루어져 있다. 엽록체 외막으로 이동하는 대부분의 단백질들은 세포질에서 합성된 후에, 소포체 또는 미토콘드리아 막으로의 부적절한 삽입(insertion)을 피하여, 특이적으로 엽록체 외막으로 삽입된다(Day and Theg, 2018). 하지만 외막을 제외한 엽록체의 다른 위치(엽록체 내부)로 이동하는 단백질들은 아미노 말단에 transit peptide라고 하는 단백질 이동 신호를 이용하여 엽록체 내부로 수송된다(Lee and Hwang, 2018).
Transit peptide는 아미노 말단에서 기능을 하는 이동 신호이고, 소포체, 핵, peroxisome 등으로 이동하는 단백질들이 가지는 이동 신호에 비해서 상대적으로 길이가 길다(대략 60~120개의 아미노산)(Bruce, 2000). 그리고 엽록체 내부로 이동한다고 알려진 수백 개가 넘는 단백질들의 transit peptide 아미노산 서열(일차구조)들을 분석해 보면 유사성이 거의 존재하지 않는다(Bhushan et al., 2006; Lee and Hwang, 2018). 더욱이, 이차구조(secondary structure)에 있어서 transit peptide들은 매우 융통성(flexibility) 있는 unstructured한 특성을 지니고 있다. 반면에 transit peptides를 인식하여 엽록체 내부로 단백질을 수송하는 기작들은 매우 한정되어 있다. 세포질에서는 heat shock protein 70(Hsp70) 또는 Hsp90 등으로 구성된 일반적인 chaperone들이 transit peptide를 인식한다고 알려져 있다(Schwenkert et al., 2011). 엽록체 외막/내막에서는 Toc(translocon at the outer envelope of chloroplasts)/Tic(translocon at the inner envelope of chloroplast)이라고 불리우는, 이미 잘 알려진 수송 단백질 복합체가 엽록체 단백질의 이중막 통과를 담당한다(Li and Chiu, 2010). 엽록체 내부 stroma에서는 엽록체 내막을 통과하여 노출된 다양한 transit peptide들을 인식하여 단백질들을 pulling하는 chaperone 단백질인 cpHsp70과 Hsp93이 공통적으로 작용한다(Li and Chiu, 2010).
그렇다면, 어떻게 이렇게 한정된 단백질 이동 경로가 매우 다양한 transit peptides를 인식하여 엽록체 단백질들을 엽록체로 이동시킬까? 본 총설논문에서는 이 다양한 transit peptide에 대한 최근의 연구 결과들을 정리하고, transit peptide들이 공통적으로 가지는 domain structure 및 sequence motifs에 대해 정리 및 고찰할 것이다.
본 론
Transit peptide를 이용한 엽록체 내부로의 단백질 이동은 여러 단계를 거쳐서 일어난다(Lee and Hwang, 2018; Li and Chiu, 2010). 먼저 세포질에서 만들어진 엽록체 단백질들은 다른 세포소기관으로의 mis-sorting을 피해 세포질을 거쳐 엽록체로 이동해야 한다(cytosolic navigation). 그 후, transit peptide를 이용하여 엽록체 외막의 Toc translocon 복합체와 결합하고, Toc translocon의 Toc75라는 수송단백질(channel)을 통하여 엽록체 외막을 통과한다. 외막을 통과한 단백질은 intermembrane space를 거친 다음, Tic translocon 복합체를 통하여 엽록체 내막을 통과하게 된다. 엽록체 단백질이 엽록체 이중막을 통과할 때, stroma에 존재하는 여러 chaperone 단백질들(Hsp93, cpHsp70)이 지닌 ATPase 활성에 의해 통과된다고 알려져 있다(Li and Chiu, 2010). 그 후, transit peptide는 stromal processing peptidase에 의해 절단(cleavage)이 된다(Richter and Lamppa, 1999). 이 때, 일부의 엽록체 내막 단백질들은 Tic translocon을 통과하는 중에 내막으로의 삽입이 이루어진다(stop-transfer 기작)(Fig. 1). 또, 다른 엽록체 내막 단백질들은 stroma로 먼저 이동한 다음 엽록체 내막으로 삽입되기도 한다(post-import 기작)(Fig. 1). Thylakoid로 이동하는 단백질들은 transit peptide가 절단된 후에 새롭게 노출된 이동 신호를 이용하여 thylakoid 내부 또는 막으로 삽입된다(Lee et al., 2017; Fig. 1).
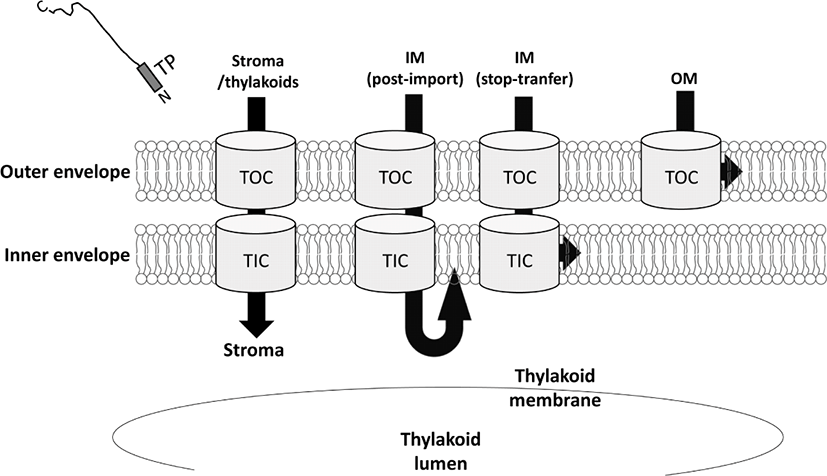
서론에서 언급하였듯이, Transit peptide들은 일차구조(primary structure)에 있어서 매우 다양한(diverse) 성격을 띄고 있다(Bhushan et al., 2006; Bruce, 2000; Lee and Hwang, 2018). 하지만, 아미노산 조성의 관점에서는 공통된 특성들도 존재한다. 첫째로 transit peptide들은 serine, threonine과 같은 hydroxylated 아미노산의 분포가 상당히 많다(Bhushan et al., 2006; Bruce, 2000; Zhang and Glaser, 2002; Zybailov et al., 2008). 하지만, 엽록체 내부로의 단백질 이동에 있어서 이 serine/ threonine의 역할은 아직 확실히 규명된 바가 없다. 왜냐하면, 이 serine/threonine 잔기들을 alanine으로 치환시킨 transit peptide를 지닌 엽록체 단백질의 경우, 엽록체로의 이동이 정상적으로 이루어졌기 때문이다(Lee et al., 2006). 반면에 serine/threonine 잔기 주위에 있는 hydrophobic한 아미노산들은 단백질이 세포질을 통과할 때 매우 중요하다는 결과를 반복해서 얻을 수 있었다(Lee et al., 2006; Lee et al., 2008; Lee et al., 2015). 그리고 미토콘드리아로 이동하는 단백질의 이동신호인 presequence의 경우에는, 비슷한 위치에 상당수의 arginine 잔기들이 존재하는데, 이 arginine들이 바로 엽록체로의 이동을 피하게 해주는 중요한 motif로 알려져 있다(Ge et al., 2014; Lee et al., 2019).
Transit peptide의 중간 부위에 존재하는 아미노산들을 조사해 보면, 음전하(negative charge)를 띄는 glutamic acid/aspartic acid들의 분포보다 양전하(positive charge)를 띄고 있는 arginine/lysine의 분포가 훨씬 많은 것을 알 수 있다(Bhushan et al., 2006; Zhang and Glaser, 2002; Zybailov et al., 2008). 그리고, 이 양전하를 지닌 아미노산들을 음전하를 지닌 아미노산으로 치환하였을 때, 엽록체로의 이동이 심각하게 저해되는 것을 확인할 수 있었다(Lee and Hwang, 2019; Razzak et al., 2017).
마지막으로, transit peptide들은 공통적으로 상당수의 proline 잔기를 지니고 있다(Bhushan et al., 2006; Lee and Hwang, 2018; Lee et al., 2018; Zybailov et al., 2008). 이 proline 잔기의 기능을 애기장대(Arabidopsis thaliana) 원형질체(protoplast) 시스템에서 규명하였다(Lee et al., 2018). 흥미롭게도 이 proline 잔기들은 여러 단계로 구성된 엽록체 단백질의 이동 및 수송과정 중에서 엽록체의 외막/내막을 통과할 때 특이적으로 작용하는 것을 알 수 있었다(Lee et al., 2018). 더욱이 transmembrane domain(TMD)를 지닌 thylakoid 막 단백질의 경우, transit peptide에 proline이 없으면 엽록체 내부로 수송되는 대신, 엽록체 외막으로의 비정상적 삽입이 이루어지는 것을 관찰할 수 있었다(Lee et al., 2018; Fig. 2). 따라서 이 proline 잔기들은 엽록체 내부 chaperone 단백질들의 역할을 도와 효율적인 수송이 일어날 수 있게 해주는 공통적인 motif로 사료된다(Fig. 2).
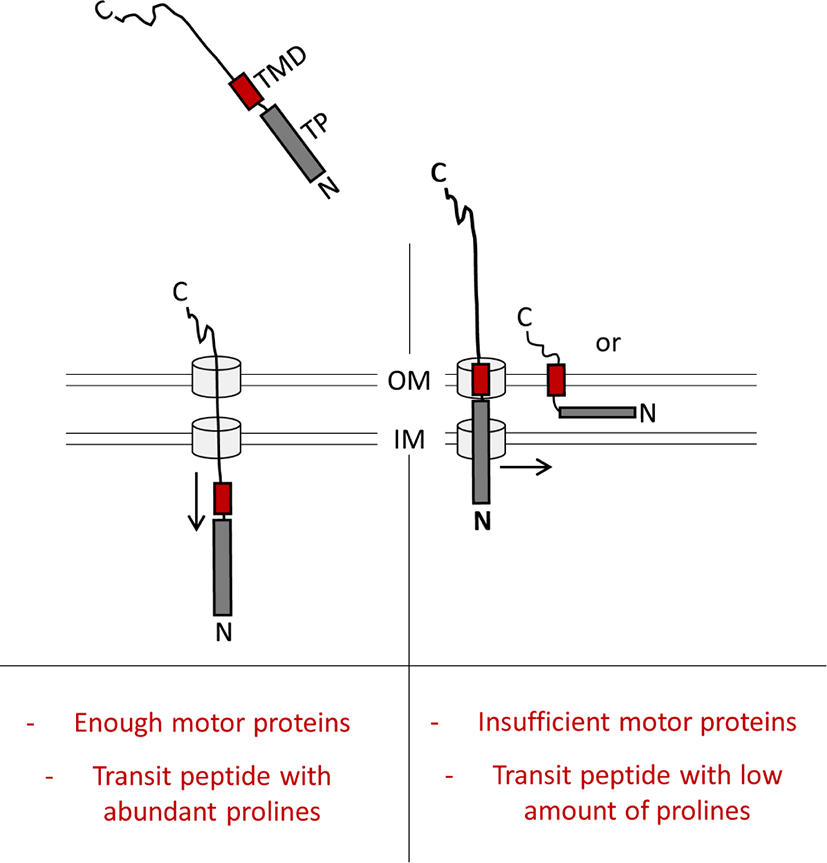
일차구조상에서의 유사성은 거의 없지만, 기능적으로 보존되어 있는 transit peptide들의 도메인 구조를 파악하기 위해서, 여러 가지의 transit peptide의 도메인을 이용한 shuffling 실험을 진행한 바 있다(Lee et al., 2009; Lee et al., 2015). 대표적인 엽록체 단백질인 Rubisco small subunit(RbcS), pyruvate dehydrogenase E1a subunit (E1a)와 chlorophyll a/b-binding protein(Cab)의 transit peptides를 여러 개의 도메인으로 나눈 다음, 서로 다른 transit peptide의 도메인을 이용하여 재조합된 hybrid transit peptide들의 기능을 살펴보았다(Lee et al., 2009; Lee et al., 2015). 흥미롭게도 hybrid transit peptide들은 엽록체 이동 신호로써의 기능을 완벽하게 보유하고 있었다(Lee et al., 2009; Lee et al., 2015). 더욱이 secretory pathway를 통해 식물 액포(vacuole)로 이동한다고 알려진 비엽록체 단백질인 carboxypeptidase Y (CPY)의 경우, 엽록체 이동에 중요한 sequence motifs가 포함된 특정 도메인을 만들어 주었을 때, 엽록체 이동신호인 transit peptide로서의 기능을 획득하게 되었다(Lee et al., 2015). 종합적으로, 이 결과들은 transit peptide들은 매우 극단적인 다양성을 지니고 있지만, 그 가운데 여러 개의 구별되는 기능을 지닌 sequence motif들과 도메인으로 구성되어 있다는 점을 시사한다.
요 약
Transit peptide들은 다양한 가운데서도 한정된 이동경로에 관여하는 단백질들에 의해 인식이 가능한, 매우 정교한 구성 원리를 지니고 있다. 요약을 하면, 아미노 말단 부위의 적절한 hydrophobicity, 중간 부위의 양전하, 전반적으로 분포된 proline 잔기, 그리고 미토콘드리아로의 부적절한 이동을 피하기 위해 아미노 말단에 arginine 존재의 회피 등을 들 수 있다(Fig. 3). 내부공생 중에 확립되었을 것으로 추정되는 다양한 transit peptide들은 이런 중요한 sequence 요소(sequence elements)들의 선별적인 조합으로 이루어졌을 것으로 추정된다(Lee et al., 2015). 본 연구주제는 엽록체를 지닌 식물의 진화와 발달을 이해하는데 많은 기여를 할 것으로 사료된다.