서 론
식량문제는 인류가 직면하고 있는 가장 큰 숙제 중 하나이다. 세계 인구는 2019년 1월 말 77억 명에 도달하였으며, 2050년 100억 명 시대를 맞이할 것으로 예상되고 있다. 이에 따라 2050년 식량 수요는 2015년 대비 35%가 증가한 연간 30억 톤이 필요할 것으로 예상되는데, 현재 경작지 규모를 증가시킬 여력이 없다는 점을 감안하면 단위 경작지당 작물 생산량을 증가시켜야 하는 난제를 풀어야 한다. 지금까지 육종 기술의 발달, GM 작물의 개발, 농약과 비료의 발달로 작물 생산량을 증가시켜 왔으나, 이러한 기술도 한계에 다다르고 있어 새로운 육종기술과 혁신적인 농업기술을 통해 보다 높은 생산성을 이뤄야 한다(이철호, 2016).
잡초 방제의 효율성 추구는 대규모 경작, 노동력 절감, 비용 절감 측면에서 반드시 필요한 요소로 지금까지 외래 유전자 도입을 통한 제초제 저항성을 획득한 유전자변형작물 개발에 크게 의지해 왔다. 2011년에는 전 세계 29개국에서 유전자변형작물을 재배하였으며, 미국은 전세계 유전자변형작물 재배면적의 43.1%인 6,900만ha를 재배하였고, 이어 브라질(19%), 아르헨티나(15%), 인도(6.5%), 캐나다(6.5%) 등의 순으로 유전자변형 작물을 재배했다. 작물로는 콩(47%), 옥수수(32%), 면화(15%), 카놀라(5%) 등으로 네 가지 작물이 전체의 99%를 차지하고 있다(변종영 et al., 2013; James, 2011). 이들 대부분의 유전자변형 작물은 제초제 저항성 작물이었다.
그러나 유전자변형 작물은 많은 국가에서 사회적 반대에 부딪히고 있고, 생태적 교란의 위험성도 제기된다. 글로벌 기업의 주도로 가공용 작물 등에 한정해서 활용되고 있으나, 식용으로 사용되기에 분명 한계를 가지게 된다. 따라서 새로운 기술의 도입을 통한 제초제 저항성 작물 개발이 요구되고 있으며, 그 대안으로 최근 각광받고 있는 유전자교정을 활용한 작물 개발이다. 특히 CRISPR Cas9 시스템을 활용하는 3세대 유전자교정 방법은 ZFN, TALLEN 등 다른 유전자교정 방법에 비해 작물 개발 기간이 매우 짧고 비용이 저렴하여 많은 연구자들이 활용하는 획기적인 시스템이다(Adli, 2018; Ran et al., 2013). 제초제 저항성 작물 제작을 위해 유전자교정 시스템을 활용하기 위해서는 타겟 유전자 발굴, guide RNA 디자인 과정을 필요로 하며, 지금까지 연구되어온 제초제 저항성 기작과 저항성 단백질 연구를 토대로 제초제 저항성 토마토 개발을 위한 타겟 단백질 및 타겟 아미노산을 본 리뷰를 통해 제시하고자 한다.
제초제에 의해 저해되는 식물 생합성 경로
제초제는 작용 기작에 따라 아미노산 합성저해와 광합성 관련 클로로필 합성저해 또는 ROS 생성 촉진 제초제 부류로 나눌 수 있다(Fig. 1). 각 제초제가 작용하는 식물체 내 생화학 반응에서 제초제는 반응물의 analogue로 작용하여 몇 가지 생합성 경로상에 있는 효소의 기능을 방해한다.
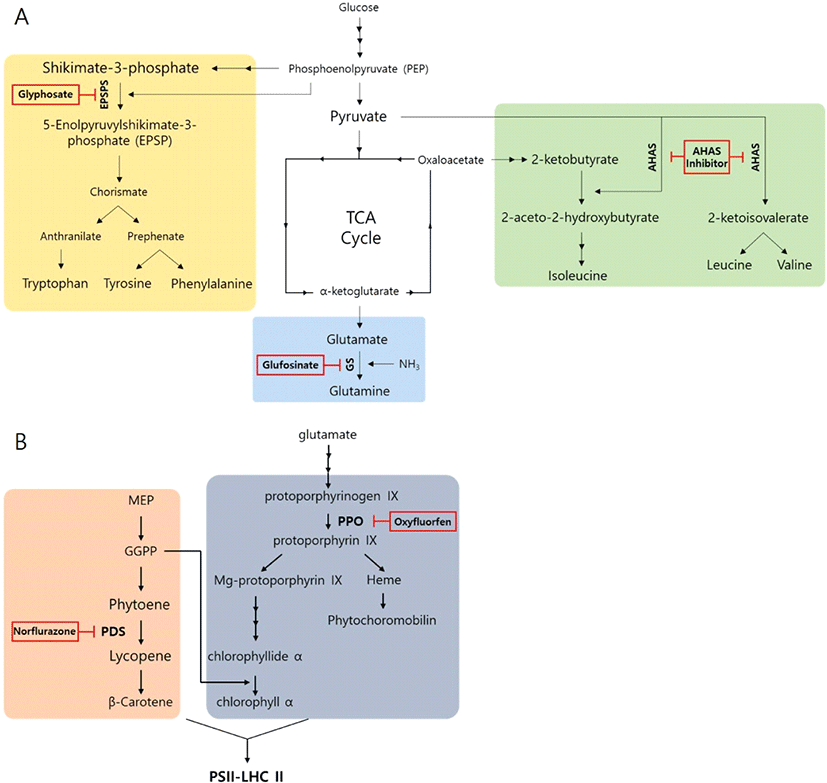
방향족 아미노산인 tryptophan, tyrosine, phenylalanine의 합성의 경로는 shikimate pathway이며, glyphosate는 shikimate pathway에서 5-enolpyruvylshikimate-3-phosphate (EPSP) 합성 과정에 필요한 5-enolpyruvylshikimate-3-phosphate synthase(EPSPS)의 효소 활성을 방해하여 방향족 아미노산의 합성을 저해한다(Fig. 1A). 따라서, glyphosate는 shikimate pathway를 통해 합성되는 식물의 필수 아미노산인 방향족 아미노산의 합성을 저해하여 잡초를 방제한다. 또한 tryptophan은 식물호르몬인 옥신(auxin)의 전구체, tyrosine은 퀴논(quinones)의 전구체, phenylalanine은 페놀릭 화합물의 전구체로 식물에 사용된다. 이들 생성물들 또한 식물의 필수 화합물로써 식물의 생장 저해를 유발한다. 구체적인 glyphosate의 작용 기작은 반응물 중 하나인 phosphoenolpyruvate (PEP)와 경쟁하여 EPSPS에 결합하여 효소반응을 방해한다(Fig. 2A; 임선형 et al., 2015).
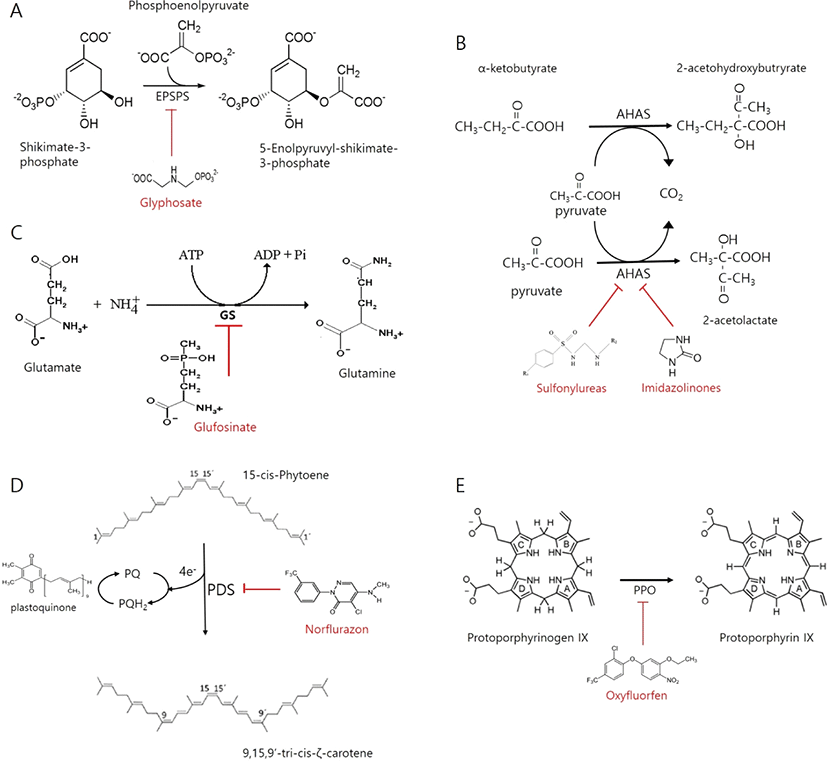
Acetohydroxyacid synthase(AHAS) 또는 acetolactate synthase(ALS)는 leucine, valine, isoleucine과 같이 branched 지방족 아미노산의 합성에 필수적인 효소이다(Fig. 1A). AHAS는 2개의 pyruvate 분자의 결합을 촉매하여 valine과 leucine의 전구체인 acetolactate를 형성한다. 또한 pyruvate와 α-ketobutyrate의 결합은 isoleucine의 전구체인 acetohydroxybutyrate를 생성한다(Fig. 2B) (Endo et al., 2013). AHAS에 의한 생합성 대사는 valine, leucine, isoleucine의 AHAS에 대한 allosteric 결합에 의해 조절된다(Endo et al., 2013). Sulfonylurea, imidazolinone, triazolopyrimidines 등과 같은 제초제에 의해 활성이 저해될 수 있다(Fig. 1A). AHAS를 저해하는 제초제 분자는 Acetolactate synthase(ALS)-FAD-thiamine pyrophosphate-Mg2+-decarboxylatedpyruvate복합체에 결합하여 두번째 pyruvate의 결합부위를 두고 서로 경쟁한다(Duke, 1990). 또 다른 AHAS 저해 제초제인 imidazolinone 또는 sulfonylurea 종류는 pyruvate와 비경쟁적인 반응을 한다. 비경쟁적이긴 하나 pyruvate는 이들 제초제가 결합하기 전에 AHAS에 결합해야 하며, 그렇지 않을 경우 효소에 대한 결합이 매우 느려진다.
Glutamine synthetase(GS)는 세포질과 색소체에서 L-glutamate를 L-glutamine으로 전환하는 과정을 통해 암모니아 동화를 촉매하는 단백질이다(Fig. 1A). Glufosinate는 GS효소를 억제시키며, 이는 식물에서 glutamine의 결핍 및 암모니아 축적을 초래할 수 있다(Fig. 2C). 이러한 암모니아의 축적은 광인산화작용을 억제하여 광합성 활동이 낮아지게 되고, 활성산소종의 과다발현, 엽록소의 파괴 등의 결과를 초래하게 된다(김진석, 2000). Glyfosinate에 의한 GS 활성 저해는 구체적으로 Glutamate에 암모니아를 전이시켜 glutamine을 생성하는 과정에서 중간물질로 γ-glutamyl phosphate를 생성하는데, glyfosinate는 γ-glutamyl phosphate와 유사한 구조를 가져 효소 결합 부위에 비가역적으로 결합하여 효소 반응을 억제한다(Hopfner et al., 1988; Kishore et al., 1988).
Phytoene desaturase(PDS)는 carotenoid 생합성 경로상에 위치하여 phytoene을 lycopene으로 변환하는 효소이다(Fig. 1B). PDS의 활성은 대표적으로 norflurazon, fluridone 등과 같은 제초제에 의해 저해된다(Fig. 2D)(김진석, 2000). Carotenoid는 광계II(Photosystem II, PSII)를 구성하는 단백질 복합체의 결합과 안정성에도 기여하여 광계II 형성에 중요한 역할을 한다고 알려져 있다(Depka et al., 1998). 또한 carotenoid는 흡수 된 빛을 chlorophyll로 전달하고, 엽록소를 분해를 억제하여 엽록체를 보호하는 것으로 알려진다(Bartels et al., 1978). PDS 저해를 통한 제초제의 작용은 크게 두 가지 방향으로 나타난다. 첫째, 성장한 잎에서는 carotenoid 결핍 시 엽록소가 파괴되어 괴사한다(Boger, 1996). 둘째, 어린잎에서 PDS가 저해되면 잎이 성장하는 동안 엽록체에 광계가 정상적으로 형성되지 못해 백화현상을 나타내며, 광합성이 불가능한 잎을 생성하게 된다. 그러므로 어린잎에서는 활성산소 발생으로 인한 산화적 스트레스 유발보다는 광합성 탈락에 의한 식물의 고사를 유도하게 된다. 따라서 식물 잎에서 PDS 저해로 유도되는 carotenoid 함량의 감소는 잎의 생육 주기에 따라 서로 다른 생장 저해 효과를 나타내게 된다.
Protoporphyrinogen oxidase(PPO)는 diphenylether (oxyfluorfen), N-phenylphthalimide(Flumioxazin), oxadiazole(Oxadiazon) 등과 같은 제초제의 작용점이다. PPO는 protogen(protoporphyrinogen IX)을 산화시켜 protoporphyrin IX을 생성한다(Fig. 1B). PPO의 억제 또는 기능적 손실은 식물체 내에서 protogen의 축적을 가져온다. 축적된 protogen은 엽록체에서 세포질로 누출되고, 이것은 산소에 의해 자발적으로 산화되어 세포질이나 원형질막에 축적된다. Proto(oxidized protogen)는 아주 강한 photosensitizer로서 빛의 존재하에 고에너지상태가 되고, 이 에너지는 산소로 전달되어 singlet oxygen을 생성한다(김진석, 2000; Matringe and Scalla, 1988; Becerril and Duke, 1989; Sandmann et al., 1990; Jacobs et al., 1991; Jacobs and Jacobs, 1993; Lee and Duke, 1994). 이러한 작용이 많아지면 식물체내 축적된 proto는 지질 과산화 및 세포 사멸을 유발하여 잎이 탈색되면서 괴사한다(Anderson et al., 1998).
따라서 제초제 저항성 작물은 일반적으로 이들 생합성 단백질을 과발현하여 야생형에 비해 상대적인 약제의 수용 능력을 키우거나, 약제에 낮은 감수성을 보이는 돌연변이 단백질을 생성하여 감수성을 낮춰주는 방법으로 생산할 수 있다. 특히 감수성이 낮은 단백질을 보유한 이끼류 돌연변이들을 분리하여 제초제 저항성에 대한 연구가 활발히 이뤄지고 있다. 유전자교정 방법을 활용하기 위해서는 내재된 유전자의 돌연변이를 유도하는 방법이 사용될 수 있으므로 이들 단백질의 아미노산 염기서열을 분석하여 기질이 결합하는 부위에서 유전자교정이 일어나도록 gRNA를 디자인하고, 발생되는 여러 종류의 교정 작물에서 제초제에 감수성이 낮은 작물을 선발할 수 있다. 이를 위해 우선적으로 토마토에서 각 제초제의 목표 단백질들의 아미노산 서열을 분석하였다(Fig. 3).
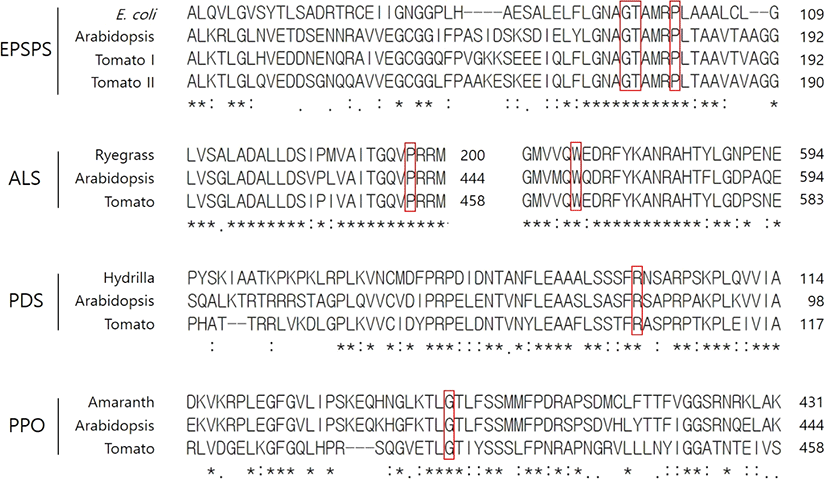
EPSPS 활성 저해 제초제인 glyphosate는 반응물 phosphoenolpyruvate(PEP)와 유사한 구조를 가지고 있어 EPSPS에 결합하여 효소 반응을 방해한다. EPSPS의 PEP에 대한 친화력을 유지하면서 glyphosate에 대한 친화력을 떨어트릴 수 있는 EPSPS를 발굴하는 것이 연구의 핵심이다. 지난 30년간 glyphosate 저항성 EPSPS 발굴을 위한 연구가 진행되었으나, 상업적으로 성공한 야생형 EPSPS는 Zea mays와 Agrobacterium tumefaciences에서 분리한 경우뿐이었다. 이후 glyphosate에 대한 친화력이 약한, 기질 특이성에 변화를 가져오는 돌연변이 EPSPS 발굴에 관한 연구가 진행되었으며, Echerichia coli를 이용한 site directed mutagenesis (SDM) 돌연변이들(T42M, G96A, T97I 그리고 P101S)이 glyphosate에 저항성을 나타냄을 발견하였다(Funke et al., 2009; Kahrizi et al., 2007). 하지만 이들 돌연변이들은 상업적으로 활용하기에는 충분하지 않았으며, Ochrobactrum anthropi에서 분리한 EPSPS의 세 개의 아미노산 변형체(N267S/P318R/M425T)를 발현한 애기장대에서 상업성에 적합한 수준의 glyphosate 저항성을 확인하였다(Tian et al., 2011). 또한 Zea mays의 EPSPS 돌연변이(T102I, P106S) 발현 옥수수도 실용적인 glyphosate 저항성 작물 생산을 가능하게 하였다(Funke et al., 2009). 최근 연구에서는 앞서 언급된 E. coli G96A/T97I/P101S 변형 EPSPS가 상업적 활용이 가능한 수준의 glyphosate의 저항성을 높인다는 보고가 있다(Feng et al., 2018). 이러한 연구를 바탕으로 토마토 EPSPS-I 유전자 교정은 G177, T178, P182(NP_001295876.1) 아미노산의 변형을 목표로 gRNA를 디자인할 수 있다(Fig. 3).
제초제를 억제하는 acetohydroxyacid synthase(AHAS)는 잡초 Lolium rigidum를 방제하는 중요한 역할을 한다(Owen et al., 2007). 야생형 L. rigidum군집에서 sulfometuron 및 imidazolinone(IMS) 제초제 imazapyr에 대해 저항성을 나타내는 개체의 분리 동정을 통해 P197A, P197R, P197Q, P197L, P197S 및 W574L 돌연변이를 분리하였고, P197, W574 위치 아미노산의 변형을 통해 제초제 저항성 L. rigidum을 얻을 수 있는 것으로 연구되었다(Yu et al., 2008). 아미노산 서열 분석을 통해 유사한 위치의 아미노산을 교정함으로써 위와 같은 종류의 제초제에 대한 감수성이 낮은 토마토를 생산하고자 한다(Fig. 3).
PDS는 carotenoid 생합성 경로를 억제하는 제초제의 주요 표적이다. Phytoene desaturase를 저해하는 제초제 fluridone에 대한 친화력이 약한 돌연변이 PDS에 관한 연구가 진행되어 시아노박테리움 Synechococcus PDS의 돌연변이가 제초제 내성을 보이는 미생물 효소를 발현했으며(Chamovitz et al., 1991), 형질 전환 담배(Nicotiana tabacum) 식물에서 발현될 때 제초제 내성을 부여하였다(Wagner et al., 2002). 또한 수생잡초 Hydrilla verticillata PDS의 R100에서 자연적으로 발생하는 돌연변이가 제초제 저항성을 부여하는 것으로 보고되었다(Michel et al., 2004). 고등식물에서 제초제 내성 유전자의 선택적 마커로서의 사용을 조사하기 위해 Hydrilla verticillata PDS의 R100을 19개의 다른 아미노산으로 대체하여 애기장대에서 발현했을 때 threonine(Thr), cysteine(Cys), alanine(Ala) 그리고 glutamine(Gln)으로의 변형 단백질이 높은 제초제 저항성을 나타내었고, 이 중 R100T 변형 단백질이 norflurazon과 fluridone에 대한 저항성이 가장 우수했다(Arias et al., 2006). 이러한 연구를 바탕으로 토마토 PDS 유전자 교정은 R100 아미노산의 변형을 목표로 gRNA를 디자인할 수 있다(Fig. 3).
PPO는 강력한 제초제 표적 중 하나다(Hao et al., 2011). 식물에서 2개의 nuclear PPO 유전자는 PPO1 (plastid-targeted, 엽록소와 heme이 합성)과 PPO2(미토콘드리아 targeted, heme이 생성)를 암호화한다 (Lermontova et al., 1997). PPO1과 PPO2를 표적으로 diphenylethers, thiadiazoles, oxadiazoles, triazolinones, N-phenyl-phthalimides, and pyrimidinediones 같은 제초제들에 대한 친화력이 약한 돌연변이 PPO에 관한 연구가 진행되었다. 담배(Nicotiana tabacum)의 PPO2 단백질의 결정 구조로부터 추론된 결과에 의하면 표지된 아미노산 Asn67, Arg98, Gly354, Leu356, Leu369, Gly370, Tyr371, Leu372 및 Phe392는 기질결합 또는 억제제 결합에 관여하는 것으로 보인다(Koch et al., 2004). Amaranthus palmeri에서 제초제 저항성 개체에서 발견된 PPO의 아미노산 위치는 Asn98, Arg128, Gly383, Leu385, Leu398, Gly399, Tyr400, Leu401 및 Phe421이며(Rangani et al., 2019), 이 결과는 담배에서의 결과와 일치했다. 두 식물체의 PPO 아미노산 서열 비교에서 30개의 아미노산 차이가 나타나는 것은 targeting peptide sequence의 존재 유무에 따른 차이이다(Fig. 3).
네 개의 제초제 저항성 단백질의 아미노산 서열 비교를 통해 토마토에서 해당 아미노산이 잘 보존되어 있음을 알 수 있었고(Fig. 3), 이를 활용해 제초제 저항성 토마토 개발이 가능함을 예측할 수 있다. 유전자교정 방법으로 개발된 제초제 저항성 작물은 기존의 제초제 저항성 작물을 대체할 수 있으며, LMO 규제에서 비교적 자유로울 수 있어 활용성이 높다. 여러 고부가가치의 기능성 작물 개발에서 제초제 저항성 형질은 실용화를 위한 기본 형질로 활용될 수 있다.